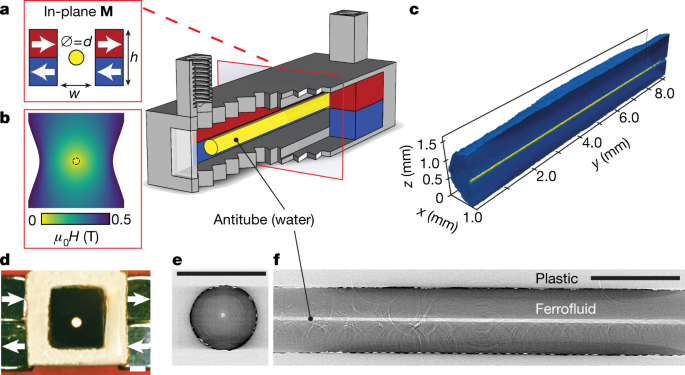
Tabeling, P. Introduction to Microfluidics (Oxford, 2005).
Mukhopadhyay, R. When microfluidic devices go bad. Anal. Chem. 77, 429A–432A (2005).
Zhao, B., Moore, J. S. & Beebe, D. J. Surface-directed liquid flow inside microchannels. Science 291, 1023–1026 (2001).
Wong, T.-S. et al. Bioinspired self-repairing slippery surfaces with pressure-stable omniphobicity. Nature 477, 443–447 (2011).
Wang, W. et al. Multifunctional ferrofluid-infused surfaces with reconfigurable multiscale topography. Nature 559, 77–82 (2018).
Leslie, D. C. et al. A bioinspired omniphobic surface coating on medical devices prevents thrombosis and biofouling. Nat. Biotechnol. 32, 1134–1140 (2014).
Forth, J. et al. Reconfigurable printed liquids. Adv. Mater. 30, 1707603 (2018).
Secchi, E. et al. Massive radius-dependent flow slippage in carbon nanotubes. Nature 537, 210–213 (2016).
Banerjee, A., Kreit, E., Liu, Y., Heikenfeld, J. & Papautsky, I. Reconfigurable virtual electrowetting channels. Lab Chip 12, 758 (2012).
Choi, K., Ng, A. H. C., Fobel, R. & Wheeler, A. R. Digital microfluidics. Annu. Rev. Anal. Chem. 5, 413–440 (2012).
Lee, W. C., Heo, Y. J. & Takeuchi, S. Wall-less liquid pathways formed with three-dimensional microring arrays. Appl. Phys. Lett. 101, 114108 (2012).
Walsh, E. J. et al. Microfluidics with fluid walls. Nat. Commun. 8, 816 (2017).
Keerthi, A. et al. Ballistic molecular transport through two-dimensional channels. Nature 558, 420–424 (2018).
Shang, L., Cheng, Y. & Zhao, Y. Emerging droplet microfluidics. Chem. Rev. 117, 7964–8040 (2017).
Zhao, W., Cheng, R., Miller, J. R. & Mao, L. Label-free microfluidic manipulation of particles and cells in magnetic liquids. Adv. Funct. Mater. 26, 3916–3932 (2016).
Coey, J. M. D., Aogaki, R., Byrne, F. & Stamenov, P. Magnetic stabilization and vorticity in submillimeter paramagnetic liquid tubes. Proc. Natl Acad. Sci. USA 106, 8811–8817 (2009).
Caravan, P., Ellison, J. J., McMurry, T. J. & Lauffer, R. B. Gadolinium(iii) chelates as MRI contrast agents: structure, dynamics, and applications. Chem. Rev. 99, 2293–2352 (1999).
Ferrand, J., Favreau, L., Joubaud, S. & Freyssingeas, E. Wetting effect on Torricelli’s law. Phys. Rev. Lett. 117, 248002 (2016).
Rosensweig, R. E. Ferrohydrodynamics (Dover, 2014).
Posocco, P. et al. Interfacial tension of oil/water emulsions with mixed non-ionic surfactants: comparison between experiments and molecular simulations. RSC Adv. 6, 4723–4729 (2016).
Owusu Apenten, R. K. & Zhu, Q.-H. Interfacial parameters for selected Spans and Tweens at the hydrocarbon–water interface. Food Hydrocoll. 10, 27–30 (1996).
Byrnes, J. et al. Hemolysis during cardiac extracorporeal membrane oxygenation: a case-control comparison of roller pumps and centrifugal pumps in a pediatric population. ASAIO J. 57, 456–461 (2011).
Omar, H. R. et al. Plasma free hemoglobin is an independent predictor of mortality among patients on extracorporeal membrane oxygenation support. PLoS ONE 10, e0124034 (2015).
Dalton, H. J. et al. Factors associated with bleeding and thrombosis in children receiving extracorporeal membrane oxygenation. Am. J. Respir. Crit. Care Med. 196, 762–771 (2017).
Valladolid, C., Yee, A. & Cruz, M. A. von Willebrand factor, free hemoglobin and thrombosis in ECMO. Front. Med. 5, 228 (2018).
Wilson, A. M. M. M. et al. Hemolysis risk after packed red blood cells transfusion with infusion pumps. Rev. Lat. Am. Enfermagem 26, e3053 (2018).
Prahl, S. Optical absorption of hemoglobin. Oregon Medical Laser Center https://omlc.org/spectra/hemoglobin/index.html (1999).
Baskin, L., Dias, V., Chin, A., Abdullah, A. & Naugler, C. in Accurate Results in the Clinical Laboratory (eds Dasgupta, A. & Sepulveda, J. L.) 19–34 (Elsevier, 2013).
Jaouen, P., Vandanjon, L. & Quéméneur, F. The shear stress of microalgal cell suspensions (Tetraselmis suecica) in tangential flow filtration systems: the role of pumps. Bioresour. Technol. 68, 149–154 (1999).
Kamaraju, H., Wetzel, K. & Kelly, W. J. Modeling shear-induced CHO cell damage in a rotary positive displacement pump. Biotechnol. Prog. 26, 1606–1615 (2010).
Vázquez-Rey, M. & Lang, D. A. Aggregates in monoclonal antibody manufacturing processes. Biotechnol. Bioeng. 108, 1494–1508 (2011).
Wang, S. et al. Shear contributions to cell culture performance and product recovery in ATF and TFF perfusion systems. J. Biotechnol. 246, 52–60 (2017).
Nesta, D. et al. Aggregation from shear stress and surface interaction: molecule-specific or universal phenomenon? Bioprocess Int. 30, 30–39 (2017).
Hejazian, M., Li, W. & Nguyen, N.-T. Lab on a chip for continuous-flow magnetic cell separation. Lab Chip 15, 959–970 (2015).
Eijkel, J. C. T. & van den Berg, A. Nanofluidics: what is it and what can we expect from it? Microfluid. Nanofluidics 1, 249–267 (2005).
Bocquet, L. & Charlaix, E. Nanofluidics, from bulk to interfaces. Chem. Soc. Rev. 39, 1073–1095 (2010).
Bocquet, L. & Tabeling, P. Physics and technological aspects of nanofluidics. Lab Chip 14, 3143–3158 (2014).
Evans, D. F. The determination of the paramagnetic susceptibility of substances in solution by nuclear magnetic resonance. J. Chem. Soc. 1959, 2003–2005 (1959).
Coey, J. M. D. Magnetism and Magnetic Materials (Cambridge Univ. Press, 2010).
Sastri, V. R., Perumareddi, J. R., Rao, V. R., Rayudu, G. V. S. & Bünzli, J.-C. G. Modern Aspects of Rare Earths and their Complexes (Elsevier, 2003).
Cugat, O., Byrne, R., McCaulay, J. & Coey, J. M. D. A compact vibrating-sample magnetometer with variable permanent magnet flux source. Rev. Sci. Instrum. 65, 3570–3573 (1994).
Wysin, G. M. Demagnetization Fields (Kansas State Univ., 2012); https://www.phys.k-state.edu/personal/wysin/notes/demag.pdf.
Furlani, E. P. Permanent Magnet and Electromechanical Devices (Academic, 2001).
Yang, Z. J., Johansen, T. H., Bratsberg, H., Helgesen, G. & Skjeltorp, A. T. Potential and force between a magnet and a bulk Y1Ba2Cu3O7−δ superconductor studied by a mechanical pendulum. Supercond. Sci. Technol. 3, 591 (1990).
Camacho, J. M. & Sosa, V. Alternative method to calculate the magnetic field of permanent magnets with azimuthal symmetry. Rev. Mex. Fis. E 59, 8–17 (2013).
Schindelin, J. et al. Fiji: an open-source platform for biological-image analysis. Nat. Methods 9, 676–682 (2012).
Daerr, A. & Mogne, A. Pendent_Drop: An ImageJ plugin to measure the surface tension from an image of a pendent drop. J. Open Res. Softw. 4, e3 (2016).
Marone, F. & Stampanoni, M. Regridding reconstruction algorithm for real-time tomographic imaging. J. Synchrotron Radiat. 19, 1029–1037 (2012).
"flow" - Google News
May 06, 2020 at 10:08PM
https://ift.tt/3c5KaxE
Liquid flow and control without solid walls - Nature.com
"flow" - Google News
https://ift.tt/2Sw6Z5O
https://ift.tt/2zNW3tO
Bagikan Berita Ini
0 Response to "Liquid flow and control without solid walls - Nature.com"
Post a Comment